An age-old problem: how CO2 adds a new layer of complexity to Germany's volcanic mystery
- alicepaine
- Feb 14, 2024
- 8 min read
Germany hides a violent past.
Situated between the cities of Trier and Aachen are the Eifel Mountains: a gently rolling plateau from which elongated mountain ridges and individual mountains rise, interspersed with patches of beech woodland and tranquil lakes. However, the Eifel Mountains are also home to the Vulkaneifel, which translates to “Volcanic Eifel” (Fig. 1).
The Vulkaneifel describes the active volcanic system that lies at the heart of the Eifel Mountains and covers an area of ~2000 km2. The field is sub-divided into ‘east’ and ‘west’. The West Eifel Volcanic Field (WEVF) contains ~240 volcanic structures including cones, tuff-rings, lava flows, and ~68 maar lakes, whereas the East Eifel Volcanic Field (EEVF) contains only ~50 volcanic structures; predominantly in the form of cones and lava-flows. However, the EEVF also contains three large calderas, one of which is Laacher See.
Approximately 12,900 years ago, a cataclysmic eruption of the Laacher See volcano shook the Vulkaneifel. With a recorded magnitude of 6.1, the eruption ejected ~6.3 km3 of magma at least 20 km into the atmosphere and blanketed an area of >1000000 km3 in fine ash, dwarfing the size of all previous eruptions known to have occurred in the Vulkaneifel since ~2 million years ago.
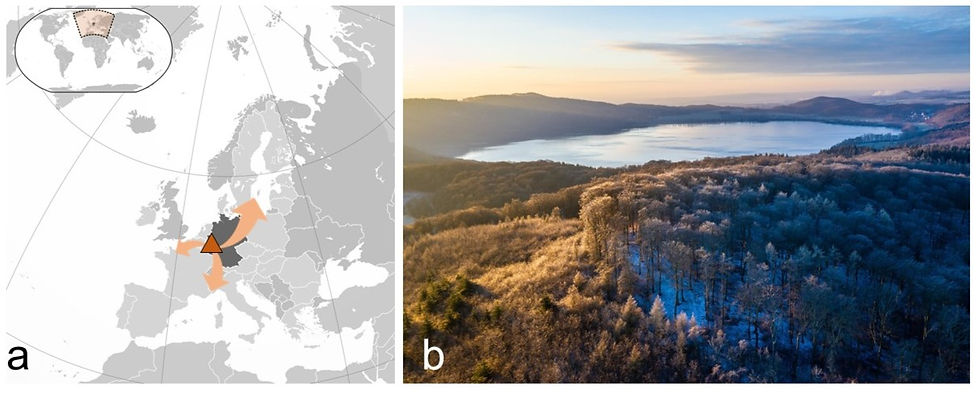
Figure 1: (a) The location of the Vulkaneifel in Germany, marked as an orange triangle. Orange arrows mark the dispersal trajectories of ash produced by the LSE eruption; (b) Photograph of the Laacher See caldera (source: https://www.eifel.info/en/a-laacher-see)
The Laacher See eruption has captured scientific attention not solely due to it’s size, but also its age.
Between ~13,000 and 11,700 years ago, the climate of Europe rapidly transitioned to unusually cold, and dry conditions. Referred to as the Younger Dryas (YD) climate anomaly, this shift temporarily reversed the gradual climatic warming occurring in the aftermath of the Last Glacial Maximum and was characterised by cold conditions across the North Atlantic and Europe, and southward shifted westerly wind belt and Intertropical Convergence Zone.
The ultimate cause of this shift remains debated, with several theories having been put forward to explain why the climate so suddenly cooled. These include a sudden ‘pulse’ of meltwater into the North Atlantic triggering a near-complete shutdown of the meridional overturning circulation system, an asteroid impact, a solar flare, and more recently, the Laacher See eruption (LSE)(1).
Volcanic eruptions have been linked to a variety of cold events across the last two millennia including the Little Ice Age, and the ‘Year without a Summer’ in 1816 CE. In 2018, Baldini and colleagues identified a link between sea level and abrupt climate change events between 120,000 and 0 years ago, whereby abrupt cooling events most typically occurred when sea levels were ~68 meters lower than today. These conditions were reached between 13,700 to 11,800 years ago, suggesting that the climate system was particularly sensitive to abrupt perturbation around the time of the LSE. From this, the authors posited that the LSE was large enough to have plausibly cooled the Earth’s surface, triggering rapid sea ice growth in the North Atlantic, slow down of the AMOC, and ultimately have resulted in a positive cooling feedback that initiated the YD.
This perspective is now supported by two pieces of proxy evidence. First, the presence of osmium ratios in cave deposits from the USA that are consistent with incorporation of volcanic aerosols and occur within uncertainty of the LSE. Second is the presence of a very large sulphur spike in ice cores from Greenland corresponding to the approximate age of the LSE, suggesting the occurrence of an exceptionally large eruption prior to the climate transition4. Taken together, both suggest that the YD was preceded by a large volcanic eruption and given its size, the LSE is a particularly strong candidate.
Despite this compelling temporal coincidence between the LSE and YD, there remains one critical problem.
We still don’t have a precise date for the Laacher See eruption. And without this date, we cannot confidently establish whether the eruption occurred before, or after, the start of the YD, and hence whether the LSE triggered this climate anomaly.
I hear you asking: why don’t we have a date?
First, we need to distinguish the difference between accuracy and precision. Accuracy is how close a given set of measurements are to their true value, whereas precision is how close the measurements are to each other. When trying to ascertain the age of prehistoric eruptions, it is impossible to know just how accurate our age estimates are, as the events were not directly observed. However, we can judge the precision of our estimated age by how similar it is to other estimates, gleaned from different techniques. For the LSE, several age estimates currently exist, each with varying degrees of uncertainty (Table 1).
Table 1: Proposed age estimates for the Laacher See eruption.
Technique | Proposed LSE Age (years) | Reference |
Lake sediment layer (varve) counting | 12,880 ± 40 | Bronk-Ramsey et al. (2015) Lane et al. (2015) |
40Ar/39Ar | 12,900 ± 560 | van den Bogaard (1995) |
Ice core SO4 | ~12,870 | Abbott et al. (2021) |
Radiocarbon | 12.934 ± 0.165 | Baales et al. (2002) |
Radiocarbon | 13,006 ± 9 | Reinig et al. (2021) |
The most recent age estimation for the LSE is that proposed by Reinig and colleagues in 2021 (Table 1). Using radiocarbon measurements from trees trapped in the ash deposits of the LSE, they present an eruption date of 13,006 ± 9 years, which is more than a century older than the previously accepted ages for the event.
Radiocarbon (14C) dating is one of the most common methods used to estimate the ages of prehistoric volcanic eruptions (Fig. 2). This can be done in one of two ways:
(1) directly - by dating organic material engulfed in ash fall or pyroclastic flow deposits
(2) indirectly - by dating organic material preserved in distal sedimentary records (lake/marine/peat) deposited below or above a geochemically distinct tephra layer.
Both approaches require the presence of organic material. Living organisms contain the same proportion of 14C as the atmosphere (or ocean in the marine realm), and this same amount of 14C within its biological material at that time remains once the organism dies. The ratio of radioactive 14C to nonradioactive 12C in its remains will gradually decrease, and because 14C decays at a known rate (half-life = 5568 years), it is possible to calculate how long it has been since a given sample stopped exchanging carbon. The older the sample, the less 14C will be left.
Figure 2: Volcanic eruptions exceeding a magnitude of 4 (>0.1 km3 ejecta) in the past 60,000 years, colour-coded according to the technique used to estimate their age. ‘Other’ refers to techniques such as 39Ar/40Ar, δ18O stratigraphy, U-Th, and zircon double-dating (ZDD).
However, acquisition of accurate 14C ages also requires that this organic material is uncontaminated. Carbon dioxide (CO2) is a particularly potent contaminant of organic material. Studies have shown that diffuse CO2 outgassing from calderas and dormant volcanic regions may actually exceed the outgassing from active volcanoes. On a local scale, organisms can incorporate measurable amounts of this degassed CO2 into their growth tissues, effectively depleting their 14C content. If these organic materials are later used to date geological events, but CO2 incorporation is not accounted for, this could result in an event age that is too old.
This problem lies at the core of our 2023 article “Possible magmatic CO2 influence on the Laacher See eruption date”. Here, we posit that this new radiocarbon date may not have sufficiently accounted for the presence of uncontaminated material, and hence that the newly proposed LSE eruption date may be ~130 years too old.
You may wonder why this is important – why should we care about a ~100-year age offset?
Well, because inaccurate dates could bias a paleoclimate archive's chronology, and subsequently ow we interpret that archive.
Age discrepancies are a significant concern for eruptions that are too old to have been recorded directly, but young enough for multi-year age differences to have a profound impact on interpretation of their impact. For example, an unrecognized offset of ~10–100 years could invalidate any correlation between a given eruption date, ice core records, and/or climatic events. In the case of the LSE, an inaccurate age could result in its exclusion as a candidate trigger of the YD; with important bearing on how we understand the long-term consequences of volcanic eruptions.
“It is worth emphasizing that we currently cannot know for certain whether the date proposed by Reinig et al. was affected by magmatic CO2. In fact, the observed increase in radiocarbon values immediately before the LSE is surprising. However, magmatic outgassing effects on radiocarbon data are still poorly understood, and we think that the evidence is strong enough, and the repercussions of an incorrect age serious enough, to warrant discussion. We suggest that until the date is independently verified, the community approach the date proposed by Reinig et al. together with the date of 12,880 ± 40 years5 to avoid possible interpretive issues.” (Baldini et al. (2023) - E2)
The LSE and its climate impact remains shrouded in mystery. As the aforementioned passage notes, it is entirely possible that the Reinig et al. LSE age was unaffected by magmatic CO2. Equally, future research may well demonstrate that the LSE did not trigger the YD. But, regardless of this uncertainty, the interdisciplinarity exhibited by Reinig and colleagues provide an exceptional example of how investment in geochronological method development will continue to prompt new questions on long-standing, intriguing questions in volcanology. This will, ultimately, lead to crucial discoveries with important bearing on how we understand volcano-climate interactions on Earth, and reconstruct the eruptive histories of unique volcanic systems.
Paper in focus: Baldini, J.U.L., Brown, R.J., Wadsworth, F.B., Paine, A.R., Campbell, J.W., Green, C.E., Mawdsley, N., Baldini, L.M. (2023) Possible magmatic CO2 influence on the Laacher See eruption date. Nature 619: E1-E2
1. Baldini, J. U. L., Brown, R. J. & Mawdsley, N. Evaluating the link between the sulfur rich Laacher See volcanic eruption and the Younger Dryas climate anomaly. Climate of the Past 14, 969–990 (2018).
2. Miller, G. H. et al. Abrupt onset of the Little Ice Age triggered by volcanism and sustained by sea-ice/ocean feedbacks. Geophysical Research Letters 39, 1–5 (2012).
3. Oppenheimer, C. Climatic, environmental and human con sequences of the largest known historic eruption: Tambora volcano (Indonesia) 1815. Progress in Physical Geography 27, 230–259 (2003).
4. Abbott, P. M. et al. Volcanic climate forcing preceding the inception of the Younger Dryas: Implications for tracing the Laacher See eruption. Quaternary Science Reviews 274, 107260 (2021).
5. Bronk Ramsey, C. et al. Improved age estimates for key Late Quaternary European tephra horizons in the RESET lattice. Quaternary Science Reviews 118, 18–32 (2015).
6. Lane, C. S. et al. The Late Quaternary tephrostratigraphy of annually laminated sediments from Meerfelder Maar, Germany. Quaternary Science Reviews 122, 192–206 (2015).
7. Baales, M. et al. Impact of the late glacial eruption of the Laacher See volcano, Central Rhineland, Germany. Quaternary Research 58, 273–288 (2002).
8. Reinig, F. et al. Precise date for the Laacher See eruption synchronizes the Younger Dryas. Nature 595, 66–69 (2021).
9. Bryson, R. U., Bryson, R. A. & Ruter, A. A calibrated radiocarbon database of late Quaternary volcanic eruptions. eEarth Discussions 1, 123–134 (2006).
10. Friedrich, W. L. et al. Santorini eruption radiocarbon dated to 1627–1600BC. Science 312, 548 (2006).
11. Pearson, C. L. et al. Annual radiocarbon record indicates 16th century BCE date for the Thera eruption. Science Advances 4, (2018).
12. Blackford, J. J., Payne, R. J., Heggen, M. P., de la Riva Caballero, A. & van der Plicht, J. Age and impacts of the caldera-forming Aniakchak II eruption in western Alaska. Quaternary Research (United States) 82, 85–95 (2014).
13. Egan, J., Staff, R. & Blackford, J. A high-precision age estimate of the Holocene Plinian eruption of Mount Mazama, Oregon, USA. The Holocene 25, 1054–1067 (2015).
14. Albert, P. G. et al. Geochemical characterisation of the Late Quaternary widespread Japanese tephrostratigraphic markers and correlations to the Lake Suigetsu sedimentary archive (SG06 core). Quaternary Geochronology 52, 103–131 (2019).
15. Johnson, F. Radiocarbon Dating: A Report on the Program to Aid in the Development of the Method of Dating. in MEMOIRS OF THE SOCIETY FOR AMERICAN ARCHAEOLOGY 1–65 (THE SOCIETY FOR AMERICAN ARCHAEOLOGY, 1951).
16. Werner, C. et al. Carbon Dioxide Emissions from Subaerial Volcanic Regions - Two Decades in Review. in Deep Carbon: past to present 188–236 (Cambridge University Press, 2019).
17. Holdaway, R. N., Duffy, B. & Kennedy, B. Evidence for magmatic carbon bias in 14C dating of the Taupo and other major eruptions. Nature Communications 9, 4110 (2018).
18. Cook, A. C., Hainsworth, L. J., Sorey, M. L., Evans, W. C. & Southon, J. R. Radiocarbon studies of plant leaves and tree rings from Mammoth Mountain, CA: a long-term record of magmatic CO2 release. Chemical Geology 177, 117–131 (2001).
19. Seiler, R. et al. Tree‐ring stable isotopes and radiocarbon reveal pre‐ and post‐eruption effects of volcanic processes on trees on Mt. Etna (Sicily, Italy). Ecohydrology 14, (2021).
20. Peiffer, L., Wanner, C. & Lewicki, J. L. Unravelling the dynamics of magmatic CO2 degassing at Mammoth Mountain, California. Earth and Planetary Science Letters 484, 318–328 (2018).
Comentarios